His encounters with invisible contagions apart, Kashmir origin American epidemiologist, Dr Ali S Khan’s book offers fascinating simplification of the complex scientific processes and the significance of the microbes to humankind. Khan asserts that not human’s, but microbes own the plant and in fact most of the human body too

We humans act like we own the planet when really it’s the microbes and the insects that run things. One way they remind us who’s in charge is by transmitting disease, often with the help of small animals, including rodents or bats. Seventy to eighty per cent of emerging infectious diseases are, in fact, zoonotic. The rest, such as drug-resistant microbes, are completely of our own making.
Not All Microbes Are Bad
This does not mean all microbes are bad. We owe them many thanks for the fermenting of wine, beer, and cheese. We’ve also harnessed them as biological production factories and even as natural insecticides. I have a very healthy respect for microbes. They are 3.5 billion years old, represent 90 per cent of all life, can produce thirty generations in a day, and have picked up the nifty trick of rapid genetic evolution by swapping advantageous pieces of genetic material through transposons and plasmids. In contrast, there is us: humans.
Modern humans are about 200,000 years old, we produce a single generation in twenty-five years, and our genetic diversity is defined by localized mating patterns.
And even we are not really a single organism at all, but a hive collective inseparable from our human microbiome. The human body contains 100 trillion cells, 90 per cent of which are microbial cells in the gut and other orifices and on its surfaces. These “passengers” come from about ten thousand different microbe species that comprise the human ecosystem.
The complex interaction with this microbiota plays an important role in keeping us healthy. At the same time, it is putatively associated with sexually transmitted infections, obesity, gastrointestinal diseases, diabetes, and rheumatoid arthritis.
We already use “good” microbes or probiotics to treat a severe form of neonatal gastrointestinal infection and prevent diarrhoea while we’re taking antibiotics. Faecal transplants from healthy donors with good microbes are the treatment of choice for patients with a severe life-threatening colon infection.
Called Clostridium difficile, this disease is associated with antibiotic use that disrupts the functioning of the good microbes in our guts. Finally, there is increasing data that early antibiotic use can lead to later obesity. Researchers now and that they can make obese mice slim by feeding them the bugs from a once obese sibling who became slim after the mouse equivalent of weight loss surgery. There are already provocative studies with faecal transplants from slim persons to those with obesity to test the link between obesity and an altered gut microbiota.
Rogue Microbes
Sometimes microbes go rogue in the hunt for new ecologic niches, akin to us moving out to the countryside when the cities get too crowded. Since the advent of modern science, we’ve fought back fairly well. Smallpox, which most likely evolved from a rodent virus, was one of the greatest scourges of humankind and undoubtedly changed the course of human history, especially in the colonization of the New World where the natives were not immune.
But, in 1980, a global effort declared that smallpox had been eradicated worldwide. So confident were we that we suspended smallpox vaccination programs. This was possible only because smallpox can’t rely on animal hosts or reservoirs, but depends for its survival entirely on person-to-person transmission. If you interrupt the transfer to a new person by finding and isolating the very last human host for the disease, or protecting the uninfected with a vaccine, then you’ve wiped it out -gone forever. Unless you’re keeping some of the virus alive in research labs, of course, which is another story. Unfortunately, with new advances in synthetic biology, the proverbial mad scientist could also readily reassemble it again from the published gene maps for nefarious purposes.
While the danger from native smallpox had been put to rest, there was concern that the ecological niche left vacant might be filled by a less deadly but still troubling disease called monkey-pox.

The Virus
Viruses exist on the border of the living and the nonliving, and there’s still some debate about which side they’re on. (I firmly believe they are alive and even collectively intelligent.)
Like a living thing, a virus has the ability to replicate, but that’s about it. Unlike mainstream life forms, it doesn’t produce all the proteins it needs to make copies of itself. That’s why it invades and hijacks other cells—often yours or mine— subverting the cytoplasmic material into making more viral proteins, rather than more of whatever cellular material would have been produced ordinarily.
The Spanish Flu
In 1918, the flu that killed 50 to 100 million people was a strain first called H1N1. Known variously as la gripe, la gripe española, or la pesadilla, it was mostly called the Spanish flu, but only because Spain was not involved in the war, which meant that it was the only European country in which the press was open about the outbreak that had been killing thousands of soldiers at the front. All the combatant nations suppressed the news to protect morale.
At some point, H1N1 made the jump from animal host to human, but at any given time multiple viruses might be invading your cells and competing to assemble the eight pieces of protein they need to create a new model of themselves. One will be better at this replication, or maybe better at getting in there in the first place, or maybe it will trigger less of an immune response. The one that does the best job of doing what viruses do will be the one to prevail against the others and make the charts at CDC and the World Health Organization.
The H1N1 from 1918 held out in the competition for forty years, replicating in humans and sometimes in pigs. But because we develop partial immunity each time a virus passes through, it became less of a threat over time, and we could think of it more like the common cold.
Then, in 1957, there was a complete shift to H2N2 prompting what became known as the Asian pandemic. While it affected mostly young children and pregnant women, it killed one to 2 million people, including 69,000 in the United States.
H2N2 remained dominant in the flu world until 1968, when H3N2 came along under the alias of “Hong Kong flu,” causing between 1 and 4 million deaths, mostly among the elderly. Absent a completely new viral overcoat, that’s the common pattern: the flu kills babies and weakened senior citizens, those who are asthmatic or who have chronic heart disease.
Then, in 1977, the old H1N1 resurfaced, probably through some sort of medical misadventure – meaning an accident in a lab, or a misguided live vaccination campaign gone astray – and it started infecting people again. Fortunately, after sixty years of cohabitation, we’d developed pretty strong immunity to it, so it was not a major pandemic.
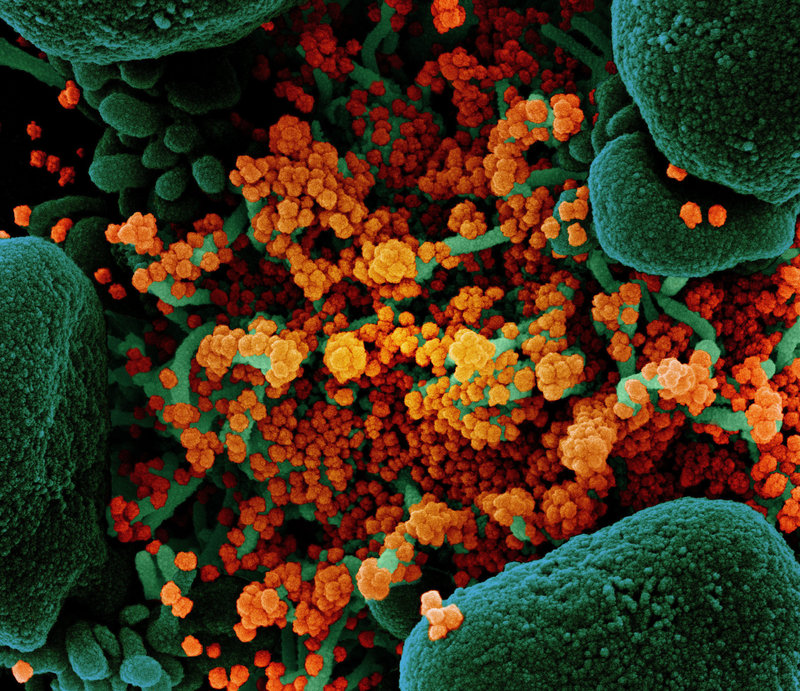
Back in 1918, when this modern progression of influenza began, all we knew about viruses was by inference. We could tell that something other than bacteria caused certain infectious diseases but that was about it. In 1892, a Russian named Dmitry Ivanovsky had poured an extract of a diseased tobacco plant through a ceramic filter fine enough to remove all bacteria, and yet the extract remained infectious. Ivanovsky thought the infectious agent might be a “toxin” caused by the bacteria. Subsequent work by others on foot and mouth disease, and on yellow fever, gave rise to descriptions like “soluble living germ” for this mysterious infectious agent. It wasn’t until improvements in optics led to better microscopes in the 1930s that true virology got underway. In 1931, the first vaccines were cultured using fertilized chicken eggs.
In the West, the vaccines given therapeutically have relied on viruses that have been killed, leaving only the residue of proteins to trigger the immune response.
In Soviet Russia, however, virologists have followed a completely different and largely isolated course: administering viruses that were alive but “attenuated,” meaning weakened.
Aside from perhaps giving more of an immunologic boost, this technique appeared advantageous in that the patient could simply inhale a tiny whiff of the vaccine rather than have an injection. Not only might this be less problematic in terms of skin reactions, but it might also be vastly cheaper for massive immunization programs, especially in developing countries.
Virologists and public health officials in the West had been wondering for quite a while if the Soviet approach made more sense. In the early 1990s, with the collapse of the USSR, we finally had a chance to compare notes, and I was the one who went over to make the comparison.
Delayed Discovery
In epidemiology, when we talk about chains of transmission – the paths a disease microbe travels from one host to another during an outbreak—one of the key questions is, will a point come when this chain becomes so long that it’s impossible to break? That is, when do you have so many human hosts harbouring a communicable disease that it no longer needs an animal reservoir at all? That’s the point when you’re no longer talking about an animal-borne disease. You’re talking about a human disease.
The fancy scientific term is the “basic reproductive rate,” which is a proxy for how infectious a disease is. If it is more than one, then people can sustain the disease indefinitely because each case is associated with at least one new person infected. Measles, for example, has the highest basic reproductive number of about fifteen, which is why you need to have extremely high vaccination rates to stop outbreaks. Influenza is about two to three, but it makes up for it with a very short incubation period, meaning the time from infection to illness.
With any new disease, it’s by calculating this number that you gain some sense of the magnitude of the problem.
In an outbreak like this, if you find that the average infected household has had fewer than one additional case of infection, then you conclude that the outbreak has peaked.
But if the disease’s reproductive rate is greater than one—if on average you’re finding more than one additional sick person per household—that’s a kind of tipping point. It means the disease is capable of sustaining itself in the community. It might even be gathering momentum.
So one of the crucial questions we needed answered was how many additional cases might be in that household. Then we would compile our results to get a big picture of what was going on.
If a virus’s reproductive rate stays above one, it can persist in a population forever. This is every microbe’s dream and every epidemiologist’s nightmare. You have examples of those microbes that stick, you have examples of those that don’t stick, and the critical factor that makes them stick. That’s a critical issue for us as we think about the next global pandemic.
Which ones get to say, “Hey, I made the jump. I never have to go back to the jungle again, slumming inside rodents.” Many of our exclusive human diseases have successfully made that jump: measles, seasonal influenza, malaria, and HIV.
Drowning In Own Fluid
The 1918 influenza likely killed by creating a “cytokine storm” in the bloodstream and lungs, cytokines being small proteins involved in signalling, as in the immune response.
When the virus infects the lung, it over-stimulates the immune system, which leads to an influx of T-cells and macrophages, cells that exist to ward off invaders. But the presence of those cells activates even more of an immune response, which stimulates the production of even more cytokines. And when you have too much of a good thing too quickly, a deadly feedback loop can start to roll, and the accumulation and concentration of immune cells—free radicals, coagulation factors, tumour necrosis factor-alpha, interleukin-1, interleukin-6, interleukin-10, and interleukin-1 receptor antagonists—can damage the tissues. When this happens in the lungs, the accumulation of immune cells can block off the airways. In other words, you drown in your own fluids.
(These disjoined passages of prose and information were excerpted from Dr Ali S Khan’s book, The Next Pandemic: On The Frontlines Against Humankind’s Gravest Dangers that Hachette Books published in 2016)